Virtual Genetics Education Centre
Epigenetics
Most people have an understanding of genetics, that our outward characteristics are specified by genes encoded by the DNA sequence in our genome. But not everything is that simple. Why is a liver cell a liver cell and not a skin cell if each of our cells contains the same DNA sequence?
There are additional factors that run in parallel to the genome that determines which sets of genes are "turned on" or are expressed and which are "turned off" or inhibited at particular times, making a liver cell a liver cell and a skin cell a skin cell; this is known as the epigenome. So, whilst the Human Genome Project provided the "blueprint for life (the sequence of bases), the epigenome will tell us how things are executed" (C. David Allis).
What is epigenetics?
Epigenetics is defined as the study of heritable changes in gene expression without a change in DNA sequence i.e. changing the phenotype without changing the genotype. In reality, this means that there is another level of genetic control in addition to a person's DNA sequence which determines which genes are turned off and which are turned off.
- Epigenetics: Changes in gene expression arising from chemical modification of DNA or histone proteins.
- Phenotype: observable outward appearance of an cell, organism or individual.
- Genotype: the genetic make up of a cell, organism or individual.
The epigenome is a series of chemical modifications that occur on DNA or specific amino acids in histone proteins that DNA is wrapped around. These act as markers which tell genes where they should be active or inactive at certain times, in the same way traffic lights change from red to green.
How is DNA packaged?
If the DNA in each cell was stretched out it would roughly be 2 metres in length- for this to be contained in the nucleus of cell, it must be compacted and packaged so that is is small enough to fit. This is a dynamic process where DNA is compacted into chromatin fibres that associate with histone and non-histone proteins (Figure 1).
Epigenetic mechanism
Figure 1: DNA is subject to multiple levels of organisation in order to be compact enough to fit into the nucleus of cells. A DNA molecule is around 2nm wide; this is associated with histones (bottom right) to create a "beads-on-a-string" 11nm wide chromatin fibre which becomes more condensed to create a 30nm wide chromatin fibre of compacted nucleosomes (bottom middle), before forming a condensed mitotic chromosome (top left) which is around 1400nm wide. (Creative Commons; National Institutes of Health)
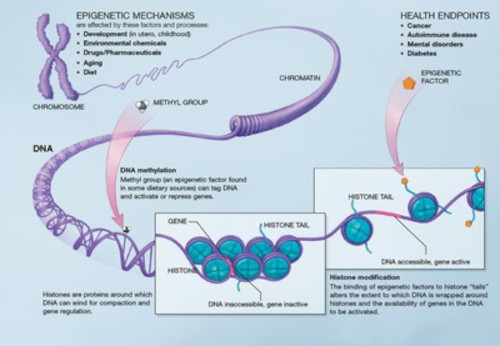
Histone proteins act like a spool that DNA can be wound around to become more compact. Initially, 146 base-pairs of DNA are wrapped 1.7 times around an octamer of core histone proteins, a H3-H4 tetramer and two H2A-H2B dimers to create a nucleosome; arrays of nucleosomes form the basic unit of chromatin (Thiagalingam et al., 2000).
Histones
Figure 2: DNA and histone proteins associated together using electrostatic interactions. DNA (sugar-phosphate backbone: orange, bases: coloured lines) wraps around the histone proteins (coloured coils in centre) whose tails protrude from the structure and are modified. (Creative Commons; Authour: Emw)
Histone proteins contain a globular head domain and a charged N-terminal tail that extends outwards from the core nucleosome structure (Figure 2) (Jenuwein and Allis, 2001). These tails are largely unstructured and it is these that are the target for epigenetic modification including acetylation, methylation and phosphorylation.
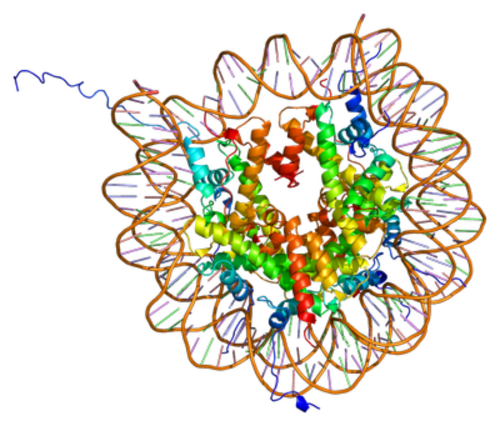
Ultimately, the chromatin fibre is further compacted to create a 30nm fibre which becomes more and more condensed at each level of packaging before forming a 1400nm wide mitotic chromosome.
Types of epigenetic modification
There are two main types of epigenetic modification:
DNA methylation
This reaction adds a methyl group to DNA and catalysed by enzymes known as DNA methyltransferases. It occurs on 5-position (C5) of cytosine nucleotides that are found next to a guanine nucleotide in the DNA sequence that are linked by a phosphate group to form a CpG dinucleotide and forms 5-methyl-cytosine. This modification acts as binding sites for other proteins which "read" the modification and recruit other and/or are associated with other proteins which can modify histones
Histone modifications
There are multiple types of histone modifications which are catalysed by a number of enzyme families; the most well characterised modifications include acetylation and methylation of histones H3 and H4 . The modifications directly alter the DNA-protein interactions to change how chromatin is structured which will alter the ability for a gene to be transcribed and expressed.
Acetylation is performed by histone acetyltransferases (HATs) which add an acetyl group to lysine amino acids (which are positively charged) in this histone tail which acts to mask the positive charge. This causes loosening of chromatin to promote gene activation (Strahl and Allis, 2000).
This is reversed by histone deacetylases (HDACs) which remove the acetyl group to unmask the positive charge, causing chromatin condensation or tightening and gene inactivation.
Methylation can occur on lysine or arginine amine acids and can occur in mono-, di- or tri-methylation events by histone methyltransferases. This mark does not substantially alter the charge of amino acids and can be associated with both gene activation and inactivation (Hawakawa and Nakayama, 2011).
- Methylation: CH3 (methyl) group added by histone methyltransfeases. Associated with gene activation and repression
- Acetylation: COCH3 (acetyl) group added by histone acetyltransferases. Associated with gene activation.
- Deacetylation: COCH3 (acetyl) group removed by histone deacetyltransferases. Associated with gene repression.
It is the modifications found on chromatin which influences the overall structure: if chromatin is loose or decondensed (euchromatin), it is in an active conformation and genes are likely to be transcribed and expressed. However, if chromatin is in a compact state (heterochromatin), it is in an inactive state, inhibiting DNA transcription and gene expression.
Epigenetics, the environment and disease
Unlike the genome which is largely static, the epigenome is much more dynamic and can respond to changes in the environment such as diet, pollution, lifestyles factors such as smoking, alcohol and stress, radiation and chemical exposure.
The mutation rate for the human genome is around 1.1x10-8 per site per generation (Roach et al., 2010). Conversely, the epigenome is significantly more flexible and can change in response to different environmental conditions.
This means that epigenetics can be linked to disease as altered epigenetic modifications can affect the timing and level of expression of particular genes so that they could be on when they should be off or off when they should be on. Normally, gene expression is a tightly regulated process so alteration of gene expression profiles may contribute directly to disease initiation and progression.
As such, epigenetics provides the missing link on how the environment can change the cell to cause disease without causing mutation which was traditionally thought as the main mechanism of causing disease.
Recent evidence shows that modifications can be passed from generation to generation in a phenomenon known as transgenerational inheritance (Bohacek and Mansuy, 2013). This means that altered gene expression profiles in a parent could be inherited by their children and grandchildren which would have direct implications for health because it means individuals could develop a disease depending on the environment their parent or grandparent was exposed to even if they weren't exposed to it themselves.
Similarly, epigenetic modifications can be associated with particular diseases such as cancer, diabetes, cardiovascular disease and obesity so inheritance of these modifications means an individual would be predisposed to the disease.
The future: epigenetic therapy?
The key to understanding disease is to thoroughly understand how epigenetic modifications are added, maintained and processed. But how can our current knowledge be used to our advantage?
As many of epigenetic modifications are reversible, this could have major implications for the development of therapeutics for disease as it may be possible to reverse abnormal modifications or potentially add beneficial modifications to change gene expression profiles.
SAHA is a histone deacetylase inhibitor used in the treatment of cutaneous T-cell lymphoma. This drug prevents the removal of acetyl groups added to histone tails which would usually cause DNA decondensation. By halting this profile, chromatin would be in a more open conformation, allowing the activation of genes.
5-azacytidine is a cytosine analogue (looks similar to the nucleotide cystosine) which is used for patients suffering from myelodysplastic syndrome (MDS). The drug acts to inhibit DNA methylation as it becomes incorporated into DNA during DNA replications, blocking DNA methyltransferase activity which can help to reactivate genes which have been silenced (Szyf, 2009).
Currently, the mechanism of action of these drugs is not entirely understood and one of the major hurdles of developing effective epigenetic therapies in the future is that there is limited understanding of the scale and specificity of epigenetic changes in many complex diseases. However, with further research into the key enzymes involved in epigenetic modifications and how to target abnormal cells specifically whilst causing minimal damage to healthy cells, more effective therapies will be determined for a wide ranges of diseases including cancer (Yoo and Jones, 2006).
Bibliography
- Bohacek, J. and Mansuy, I.M., 2013. Epigenetic inheritance of disease and disease risk.
- Hayakawa, T. and Nakayama, J., 2011. Physiological roles of Class I HDAC complex and histone demethylase.
- Jenuwein, T. and Allis, C.D., 2001. Translating the histone code.
- Roach, J.C. et al., 2010. Analysis of Genetic Inheritance in a Family Quartet by Whole-Genome Sequencing.
- Strahl, B.D. ad Allis, C.D., 2000. The language of covalent histone modifications.
- Thiagalingam, S. et al., 2000. Histone deacetylases: unique players in shaping the epigenetic histone code.
- Szyf, M., 2009. Epigenetics, DNA Methylation and Chromatin Modifying Drugs.
- Yoo and Jones, 2006. Epigenetic therapy of cancer: past, present and future.
What is epigenetics?
Epigenetics is defined as the study of heritable changes in gene expression without a change in DNA sequence i.e. changing the phenotype without changing the genotype. In reality, this means that there is another level of genetic control in addition to a person's DNA sequence which determines which genes are turned off and which are turned off.
- Epigenetics: Changes in gene expression arising from chemical modification of DNA or histone proteins.
- Phenotype: observable outward appearance of an cell, organism or individual.
- Genotype: the genetic make up of a cell, organism or individual.
The epigenome is a series of chemical modifications that occur on DNA or specific amino acids in histone proteins that DNA is wrapped around. These act as markers which tell genes where they should be active or inactive at certain times, in the same way traffic lights change from red to green.
How is DNA packaged?
If the DNA in each cell was stretched out it would roughly be 2 metres in length- for this to be contained in the nucleus of cell, it must be compacted and packaged so that is is small enough to fit. This is a dynamic process where DNA is compacted into chromatin fibres that associate with histone and non-histone proteins.
DNA is subject to multiple levels of organisation in order to be compact enough to fit into the nucleus of cells. A DNA molecule is around 2nm wide; this is associated with histones (bottom right) to create a "beads-on-a-string" 11nm wide chromatin fibre which becomes more condensed to create a 30nm wide chromatin fibre of compacted nucleosomes (bottom middle), before forming a condensed mitotic chromosome (top left) which is around 1400nm wide. (Creative Commons; National Institutes of Health)
Histone proteins act like a spool that DNA can be wound around to become more compact. Initially, 146 base-pairs of DNA are wrapped 1.7 times around an octamer of core histone proteins, a H3-H4 tetramer and two H2A-H2B dimers to create a nucleosome; arrays of nucleosomes form the basic unit of chromatin (Thiagalingam et al., 2000).
DNA and histone proteins associated together using electrostatic interactions. DNA (sugar-phosphate backbone: orange, bases: coloured lines) wraps around the histone proteins (coloured coils in centre) whose tails protrude from the structure and are modified. (Creative Commons; Authour: Emw)
Histone proteins contain a globular head domain and a charged N-terminal tail that extends outwards from the core nucleosome structure (Figure 2) (Jenuwein and Allis, 2001). These tails are largely unstructured and it is these that are the target for epigenetic modification including acetylation, methylation and phosphorylation.
Ultimately, the chromatin fibre is further compacted to create a 30nm fibre which becomes more and more condensed at each level of packaging before forming a 1400nm wide mitotic chromosome.
Types of epigenetic modification
There are two main types of epigenetic modification:
- DNA methylation
- This reaction adds a methyl group to DNA and catalysed by enzymes known as DNA methyltransferases. It occurs on 5-position (C5) of cytosine nucleotides that are found next to a guanine nucleotide in the DNA sequence that are linked by a phosphate group to form a CpG dinucleotide and forms 5-methyl-cytosine. This modification acts as binding sites for other proteins which "read" the modification and recruit other and/or are associated with other proteins which can modify histones
- Histone modifications
- There are multiple types of histone modifications which are catalysed by a number of enzyme families; the most well characterised modifications include acetylation and methylation of histones H3 and H4 . The modifications directly alter the DNA-protein interactions to change how chromatin is structured which will alter the ability for a gene to be transcribed and expressed.
- Acetylation is performed by histone acetyltransferases (HATs) which add an acetyl group to lysine amino acids (which are positively charged) in this histone tail which acts to mask the positive charge. This causes loosening of chromatin to promote gene activation (Strahl and Allis, 2000).
- This is reversed by histone deacetylases (HDACs) which remove the acetyl group to unmask the positive charge, causing chromatin condensation or tightening and gene inactivation.
- Methylation can occur on lysine or arginine amine acids and can occur in mono-, di- or tri-methylation events by histone methyltransferases. This mark does not substantially alter the charge of amino acids and can be associated with both gene activation and inactivation (Hawakawa and Nakayama, 2011).
Methylation
CH3 (methyl) group added by histone methyltransfeases. Associated with gene activation and repression.
Acetylation
COCH3 (acetyl) group added by histone acetyltransferases. Associated with gene activation.
Deacetylation
COCH3 (acetyl) group removed by histone deacetyltransferases. Associated with gene repression.
It is the modifications found on chromatin which influences the overall structure: if chromatin is loose or decondensed (euchromatin), it is in an active conformation and genes are likely to be transcribed and expressed. However, if chromatin is in a compact state (heterochromatin), it is in an inactive state, inhibiting DNA transcription and gene expression.
Epigenetics, the environment and disease
Unlike the genome which is largely static, the epigenome is much more dynamic and can respond to changes in the environment such as diet, pollution, lifestyles factors such as smoking, alcohol and stress, radiation and chemical exposure.
The mutation rate for the human genome is around 1.1x10-8 per site per generation (Roach et al., 2010). Conversely, the epigenome is significantly more flexible and can change in response to different environmental conditions.
This means that epigenetics can be linked to disease as altered epigenetic modifications can affect the timing and level of expression of particular genes so that they could be on when they should be off or off when they should be on. Normally, gene expression is a tightly regulated process so alteration of gene expression profiles may contribute directly to disease initiation and progression.
As such, epigenetics provides the missing link on how the environment can change the cell to cause disease without causing mutation which was traditionally thought as the main mechanism of causing disease.
Recent evidence shows that modifications can be passed from generation to generation in a phenomenon known as transgenerational inheritance (Bohacek and Mansuy, 2013). This means that altered gene expression profiles in a parent could be inherited by their children and grandchildren which would have direct implications for health because it means individuals could develop a disease depending on the environment their parent or grandparent was exposed to even if they weren't exposed to it themselves.
Similarly, epigenetic modifications can be associated with particular diseases such as cancer, diabetes, cardiovascular disease and obesity so inheritance of these modifications means an individual would be predisposed to the disease.
The future: epigenetic therapy?
The key to understanding disease is to thoroughly understand how epigenetic modifications are added, maintained and processed. But how can our current knowledge be used to our advantage?
As many of epigenetic modifications are reversible, this could have major implications for the development of therapeutics for disease as it may be possible to reverse abnormal modifications or potentially add beneficial modifications to change gene expression profiles.
- SAHAis a histone deacetylase inhibitor used in the treatment of cutaneous T-cell lymphoma. This drug prevents the removal of acetyl groups added to histone tails which would usually cause DNA decondensation. By halting this profile, chromatin would be in a more open conformation, allowing the activation of genes.
- 5-azacytidine is a cytosine analogue (looks similar to the nucleotide cystosine) which is used for patients suffering from myelodysplastic syndrome (MDS). The drug acts to inhibit DNA methylation as it becomes incorporated into DNA during DNA replications, blocking DNA methyltransferase activity which can help to reactivate genes which have been silenced (Szyf, 2009).
Currently, the mechanism of action of these drugs is not entirely understood and one of the major hurdles of developing effective epigenetic therapies in the future is that there is limited understanding of the scale and specificity of epigenetic changes in many complex diseases. However, with further research into the key enzymes involved in epigenetic modifications and how to target abnormal cells specifically whilst causing minimal damage to healthy cells, more effective therapies will be determined for a wide ranges of diseases including cancer (Yoo and Jones, 2006).
Bibliography
- Hayakawa, T. and Nakayama, J., 2011. Physiological roles of Class I HDAC complex and histone demethylase.
- Jenuwein, T. and Allis, C.D., 2001. Translating the histone code.
- Roach, J.C. et al., 2010. Analysis of Genetic Inheritance in a Family Quartet by Whole-Genome Sequencing.
- Strahl, B.D. ad Allis, C.D., 2000. The language of covalent histone modifications.
- Thiagalingam, S. et al., 2000. Histone deacetylases: unique players in shaping the epigenetic histone code.
- Szyf, M., 2009. Epigenetics, DNA Methylation and Chromatin Modifying Drugs.
- Yoo and Jones, 2006. Epigenetic therapy of cancer: past, present and future.